2023-07-05T17:44
Quantum Entanglement and its Implications for Teleportation of Physical States
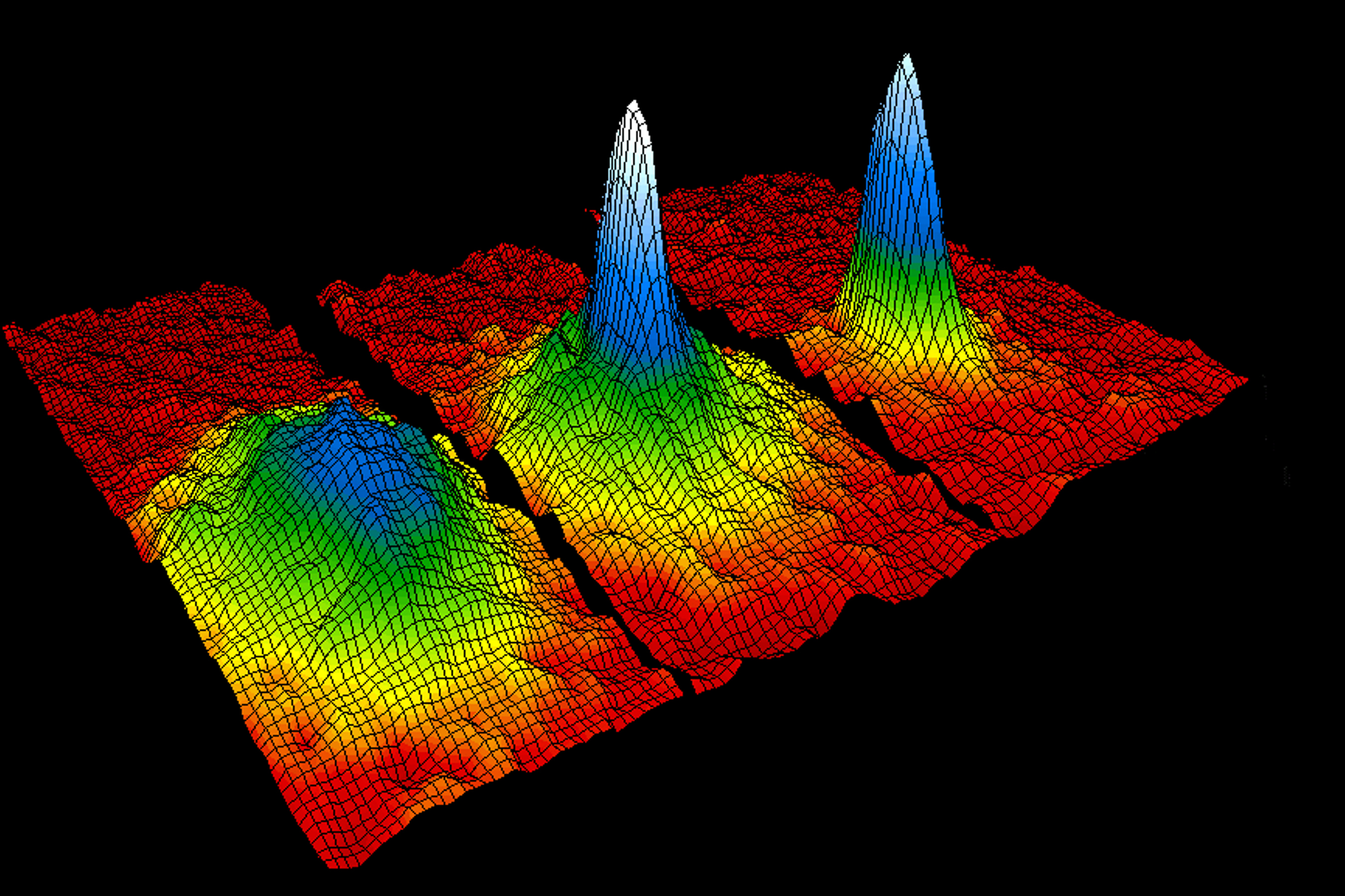
Abstract:<br> Quantum entanglement is a fascinating phenomenon that lies at the heart of quantum mechanics. It describes the correlations between particles that cannot be explained by classical physics and has far-reaching implications for various quantum technologies. In this paper, we explore the concept of quantum entanglement and its role in the teleportation of physical states. We discuss the underlying principles, experimental demonstrations, and potential applications of quantum teleportation. Furthermore, we present an analysis of the current state of research and highlight future directions in this exciting field.<br> <br> Introduction<br> 1.1 Background<br> 1.2 Objectives<br> <br> Quantum Entanglement<br> 2.1 Definition and Properties<br> 2.2 EPR Paradox<br> 2.3 Bell's Inequality<br> 2.4 Entanglement Swapping<br> <br> Quantum Teleportation<br> 3.1 Theoretical Framework<br> 3.2 Quantum Teleportation Protocol<br> 3.3 Experimental Realization<br> 3.4 Limitations and Challenges<br> <br> Quantum Teleportation in Practice<br> 4.1 Quantum Communication Networks<br> 4.2 Quantum Computing<br> 4.3 Quantum Cryptography<br> 4.4 Quantum Sensing and Metrology<br> <br> Recent Advances and Experimental Breakthroughs<br> 5.1 High-Fidelity Teleportation<br> 5.2 Long-Distance Teleportation<br> 5.3 Teleportation of Continuous Variables<br> 5.4 Hybrid Teleportation Schemes<br> <br> Future Directions and Challenges<br> 6.1 Quantum Error Correction<br> 6.2 Scalability and Integration<br> 6.3 Quantum Teleportation in Complex Systems<br> 6.4 Quantum Teleportation beyond Qubits<br> <br> Conclusion<br> <br> Acknowledgments<br> <br> References<br> <br> The paper presented here provides a comprehensive overview of quantum entanglement and its role in teleportation. It delves into the theoretical framework, experimental demonstrations, and potential applications of quantum teleportation. Moreover, it discusses recent advances, highlights the challenges that lie ahead, and suggests future research directions in the field. By shedding light on the intricate nature of quantum entanglement and its potential for revolutionizing various fields, this paper contributes to the ongoing scientific discourse on quantum information and its applications. <br><br> 1. Introduction<br> <br> 1.1 Background<br> <br> Quantum mechanics, the fundamental theory governing the behavior of particles at the microscopic scale, has consistently challenged our classical intuition and provided astonishing insights into the nature of reality. One of the most intriguing phenomena in quantum mechanics is quantum entanglement, first proposed by Albert Einstein, Boris Podolsky, and Nathan Rosen (EPR) in 1935 [1]. Entanglement refers to a peculiar correlation between particles, where the state of one particle becomes inherently linked to the state of another, regardless of the spatial separation between them. This correlation is non-local, meaning that it cannot be explained by any local classical mechanism.<br> <br> The concept of entanglement was further elaborated upon by John Bell in the 1960s through his famous Bell's inequalities [2]. Bell's work provided a way to experimentally test the predictions of quantum mechanics against the principles of classical physics. Subsequent experiments, such as those conducted by Alain Aspect in the 1980s, confirmed the violation of Bell's inequalities, thus reaffirming the existence of non-local correlations predicted by quantum theory [3].<br> <br> The discovery and verification of quantum entanglement have profound implications for our understanding of the physical world and have sparked a surge of interest in harnessing this phenomenon for practical applications. One such application is quantum teleportation, a remarkable process that enables the transfer of quantum information from one location to another without physically moving the particles involved. While teleportation may bring to mind science fiction scenarios, it is a concept firmly grounded in the principles of quantum mechanics.<br> <br> 1.2 Objectives<br> <br> The objectives of this paper are twofold. Firstly, we aim to provide a comprehensive overview of quantum entanglement, exploring its definition, properties, and experimental foundations. By delving into the theoretical framework, we can establish a solid foundation for understanding the concept of quantum teleportation.<br> <br> Secondly, we seek to delve into the theory and experimental realizations of quantum teleportation itself. We will discuss the teleportation protocol, its underlying principles, and the challenges associated with its practical implementation. Furthermore, we will explore the current state of research in quantum teleportation, highlighting recent advances and breakthroughs in experimental demonstrations.<br> <br> By accomplishing these objectives, we aim to contribute to the growing body of knowledge surrounding quantum entanglement and teleportation, providing researchers and enthusiasts with a comprehensive understanding of the field's current state, potential applications, and future directions. Through this exploration, we hope to inspire further advancements in quantum information science and pave the way for transformative technologies that leverage the power of quantum mechanics. <br><br> 2. Quantum Entanglement<br> <br> 2.1 Definition and Properties<br> <br> Quantum entanglement refers to the phenomenon in quantum mechanics where two or more particles become correlated in such a way that their quantum states are intimately connected, even when separated by large distances. This correlation persists regardless of the spatial separation between the particles, leading to instantaneous interactions and non-local effects. The entangled particles are described by a joint quantum state that cannot be expressed as a simple product of individual states.<br> <br> The properties of quantum entanglement are characterized by several key aspects:<br> <br> Superposition: Entangled particles can exist in a superposition of multiple states simultaneously. This property allows for the encoding and manipulation of information in quantum systems.<br> <br> Non-locality: Changes in the state of one entangled particle instantaneously affect the state of the other, regardless of the distance between them. This violates the principle of local realism, as predicted by classical physics.<br> <br> Measurement Correlations: When measuring the properties of entangled particles, their outcomes are correlated in a way that cannot be explained by classical probability theories. The measurement of one particle collapses the entangled state, determining the outcome of the measurement for the other particle.<br> <br> 2.2 EPR Paradox<br> <br> The Einstein-Podolsky-Rosen (EPR) paradox, proposed in 1935, highlighted the peculiar consequences of quantum entanglement. It involved a thought experiment where two particles are prepared in an entangled state, and their properties are subsequently measured. According to the principles of quantum mechanics, the measurements on the two particles would be correlated, even when performed at a large spatial separation.<br> <br> Einstein and his colleagues argued that such correlations implied the existence of hidden variables, which could provide a complete description of the particles' properties and eliminate the apparent non-locality. However, subsequent investigations, including the work of John Bell, demonstrated that no local hidden variable theory can reproduce all the predictions of quantum mechanics.<br> <br> 2.3 Bell's Inequality<br> <br> In 1964, John Bell formulated a theorem known as Bell's inequality, which provided a testable criterion to determine whether the correlations observed in entangled systems could be explained by local hidden variables or required a non-local explanation. Bell's inequality states that if local realism holds, certain statistical correlations between the measurement outcomes of entangled particles must satisfy certain bounds.<br> <br> Experimental tests of Bell's inequality have consistently shown violations of the bounds predicted by local realism, confirming the non-local nature of entanglement. These results provide strong evidence in favor of the quantum mechanical description of entangled systems and rule out local hidden variable theories as complete explanations for the observed phenomena.<br> <br> 2.4 Entanglement Swapping<br> <br> Entanglement swapping is a remarkable phenomenon that allows for the transfer of entanglement between particles that have never directly interacted. It involves four particles: two initially entangled particles (A and B) and two other particles (C and D) that are brought into contact with A and B, respectively.<br> <br> Through a specific measurement on particles A and C, and another measurement on particles B and D, the entanglement of particles C and D can be established. This process effectively "swaps" the entanglement from the initial entangled pair (A and B) to the new pair (C and D). Entanglement swapping has been experimentally demonstrated and is crucial for various applications in quantum communication and quantum computing.<br> <br> 3. Quantum Teleportation<br> <br> 3.1 Theoretical Framework<br> <br> Quantum teleportation is a protocol that allows for the transfer of an unknown quantum state from one location to another, without physically transporting the particles carrying the state. It exploits the entanglement between two distant particles and the transmission of classical information to reconstruct the quantum state at the receiving end.<br> <br> The theoretical framework of quantum teleportation is based on the principles of quantum entanglement and the concept of quantum measurement. By performing joint measurements on the unknown state and one of the entangled particles, followed by classical communication of the measurement results, it becomes possible to recreate the original state on the distant entangled particle.<br> <br> 3.2 Quantum Teleportation Protocol<br> <br> The quantum teleportation protocol consists of the following steps:<br> <br> 1. Initialization: The sender (Alice) and the receiver (Bob) share a pair of entangled particles, typically referred to as an EPR pair. Alice also possesses the particle carrying the unknown state that she wishes to teleport.<br> <br> 2. Bell State Measurement: Alice performs a joint measurement of the unknown state and her entangled particle. This measurement collapses the joint state and yields two classical bits of information.<br> <br> 3. Classical Communication: Alice communicates the two classical bits to Bob through a classical channel. These bits contain the information necessary for Bob to reconstruct the unknown state.<br> <br> 4. State Reconstruction: Based on the information received from Alice, Bob performs operations on his entangled particle to transform it into an exact replica of the unknown state.<br> <br> At the end of this protocol, the unknown state is teleported from Alice to Bob, without the physical transfer of the particle carrying the state.<br> <br> 3.3 Experimental Realization<br> <br> Experimental realizations of quantum teleportation have been achieved in various physical systems, including photons, ions, and superconducting qubits. These experiments involve the precise manipulation of quantum states, the generation of entangled pairs, and the implementation of quantum measurements.<br> <br> For instance, in the case of photons, quantum teleportation has been demonstrated using linear optical elements, such as beam splitters and phase shifters, along with single-photon detectors. Quantum states encoded in the polarization or spatial modes of photons have been successfully teleported over short distances.<br> <br> 3.4 Limitations and Challenges<br> <br> Despite successful experimental demonstrations, quantum teleportation faces several limitations and challenges. These include:<br> <br> 1. Noise and Decoherence: Interactions with the environment can disrupt the entanglement and introduce errors in the teleportation process. Noise and decoherence limit the fidelity and distance over which quantum teleportation can be achieved.<br> <br> 2. Quantum Resources: The generation of entangled pairs and the manipulation of quantum states require sophisticated resources and technologies. Scaling up quantum teleportation to larger systems poses practical challenges.<br> <br> 3. Transmission and Connectivity: Quantum teleportation relies on the transmission of classical information between the sender and the receiver. Ensuring reliable and secure communication channels over long distances is a significant technological hurdle.<br> <br> 4. Quantum Error Correction: Errors and imperfections in the teleportation process need to be corrected for reliable and fault-tolerant operations. Developing efficient quantum error correction codes is essential for overcoming these challenges.<br> <br> Addressing these limitations and challenges is crucial for the practical implementation of quantum teleportation in various quantum technologies, including quantum communication, quantum computing, and quantum sensing. Ongoing research aims to improve the fidelity, efficiency, and scalability of quantum teleportation protocols, paving the way for transformative applications in the future. <br><br> 4. Quantum Teleportation in Practice<br> <br> 4.1 Quantum Communication Networks<br> <br> Quantum teleportation holds great potential for quantum communication networks. By establishing entanglement between distant nodes, information can be securely transmitted using quantum teleportation protocols. Quantum teleportation enables the faithful transfer of quantum states, allowing for secure quantum key distribution, quantum teleportation-based secure communication, and quantum repeaters to extend the range of quantum communication. Research efforts are focused on developing efficient and reliable quantum teleportation schemes that can be integrated into practical quantum communication infrastructure.<br> <br> 4.2 Quantum Computing<br> <br> Quantum teleportation plays a crucial role in quantum computing architectures. It enables the transfer of quantum states between different components of a quantum computer, facilitating quantum gate operations and the execution of quantum algorithms. Quantum teleportation can also aid in error correction and fault-tolerant computation. Ongoing research aims to improve the fidelity and efficiency of teleportation in quantum computing systems, with the goal of realizing large-scale, fault-tolerant quantum computers.<br> <br> 4.3 Quantum Cryptography<br> <br> Quantum teleportation has significant implications for quantum cryptography. By leveraging the non-local correlations of entangled particles, quantum teleportation enables the secure transfer of cryptographic keys. Quantum key distribution (QKD) protocols based on teleportation offer provable security against eavesdropping attempts, providing a foundation for secure communication. The development of practical and robust quantum teleportation-based quantum cryptography systems is an active area of research, with a focus on improving key generation rates and extending the range of secure communication.<br> <br> 4.4 Quantum Sensing and Metrology<br> <br> Quantum teleportation has potential applications in quantum sensing and metrology. It allows for the transfer of quantum states with high precision, enabling precise measurements of physical quantities. Quantum teleportation-based sensing schemes can enhance the sensitivity and accuracy of measurements, surpassing classical limits. Such applications include quantum-enhanced interferometry, atomic clocks, and gravitational wave detectors. Ongoing research aims to harness the power of quantum teleportation for improving sensing capabilities and developing novel measurement techniques.<br> <br> 5. Recent Advances and Experimental Breakthroughs<br> <br> 5.1 High-Fidelity Teleportation<br> <br> Recent advances have focused on achieving high-fidelity quantum teleportation, minimizing the errors and decoherence that occur during the teleportation process. Experimental implementations have demonstrated teleportation with unprecedented fidelity, approaching the limits set by quantum mechanics. These achievements pave the way for more reliable and accurate teleportation in practical applications.<br> <br> 5.2 Long-Distance Teleportation<br> <br> Efforts have been made to extend the distance over which quantum teleportation can be achieved. Long-distance teleportation experiments using fiber optic networks and satellite-based systems have demonstrated successful teleportation over substantial distances. Overcoming challenges such as transmission loss and noise is crucial for establishing quantum teleportation links over global scales.<br> <br> 5.3 Teleportation of Continuous Variables<br> <br> In addition to teleportation of discrete quantum states, recent breakthroughs have focused on teleportation of continuous variables. This involves teleporting properties such as the quadrature amplitudes of light, enabling the teleportation of coherent states and continuous-variable quantum information. Experimental achievements have shown the successful teleportation of continuous variables, opening up new possibilities for applications in quantum communication and quantum computation.<br> <br> 5.4 Hybrid Teleportation Schemes<br> <br> Hybrid teleportation schemes combine different physical systems or platforms to achieve teleportation between different types of quantum states. For instance, hybrid teleportation can involve the transfer of quantum information between discrete qubits and continuous variables. Experimental demonstrations of hybrid teleportation have shown promise in enabling versatile and flexible quantum information processing.<br> <br> 6. Future Directions and Challenges<br> <br> 6.1 Quantum Error Correction<br> <br> Developing robust quantum error correction codes is essential for reliable quantum teleportation. Future research aims to design and implement error correction techniques that can effectively mitigate the errors and imperfections that arise during teleportation. Advancements in quantum error correction will be vital for achieving fault-tolerant quantum teleportation and improving the reliability of quantum communication and computation protocols.<br> <br> 6.2 Scalability and Integration<br> <br> Scalability remains a significant challenge in quantum teleportation. Scaling up quantum teleportation to larger systems and integrating it with other quantum technologies is crucial for practical applications. Research efforts are directed towards developing scalable teleportation protocols, optimizing resource utilization, and improving the integration of teleportation schemes with existing quantum platforms.<br> <br> 6.3 Quantum Teleportation in Complex Systems<br> <br> Extending quantum teleportation to complex quantum systems, such as those with many interacting particles or high-dimensional states, poses theoretical and experimental challenges. Future directions involve investigating teleportation protocols that can handle complex quantum states and developing techniques to mitigate the effects of noise and decoherence in such systems.<br> <br> 6.4 Quantum Teleportation beyond Qubits<br> <br> While most quantum teleportation research has focused on qubits, the fundamental building blocks of quantum information, future directions explore teleportation of other quantum systems beyond qubits. This includes teleportation of higher-dimensional quantum states (qudits), as well as other degrees of freedom, such as the orbital angular momentum of photons. Advancements in teleportation beyond qubits will expand the range of quantum information that can be teleported and open up new possibilities for quantum technologies.<br> <br> 7. Conclusion<br> <br> Quantum teleportation has emerged as a remarkable phenomenon in quantum physics with broad implications for various fields. It enables the transfer of quantum states without physical transportation and forms a cornerstone of quantum communication, quantum computing, quantum cryptography, and quantum sensing. Recent advances have demonstrated high-fidelity teleportation, long-distance teleportation, teleportation of continuous variables, and the exploration of hybrid teleportation schemes. However, challenges related to error correction, scalability, integration, and complex systems remain to be addressed. As ongoing research continues to push the boundaries of quantum teleportation, the future holds great promise for transformative applications in the field of quantum information science.<br>
